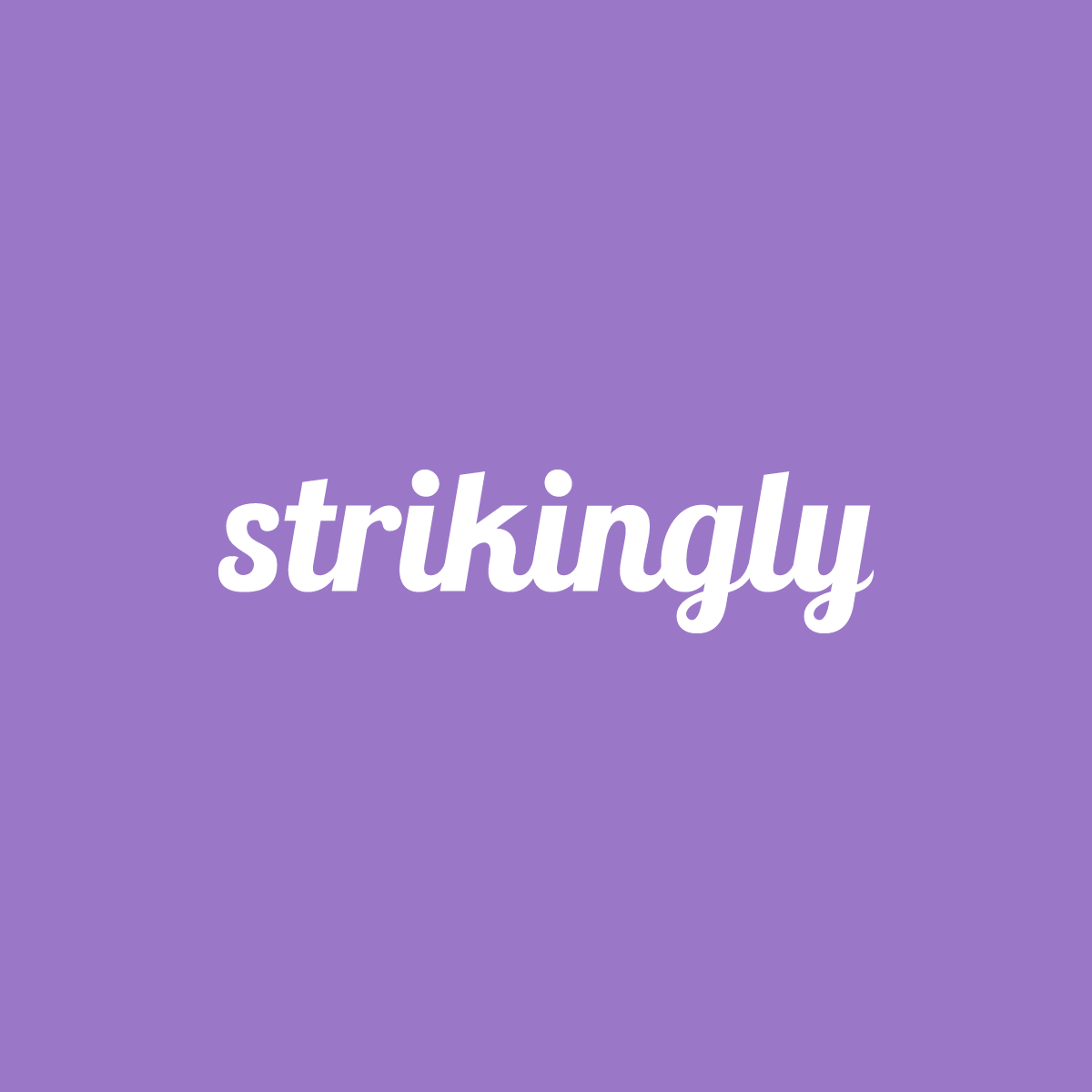
Development Process for an Engineered Plastic-Degrading Solution
A Step-by-Step Guide to Creating a Genetically Engineered Saccharomyces Cerevisiae for PET Degradation
1Genetic Segregation, Sample Collection, and DNA Extraction:
- Sample Collection: Collect samples of plastic-degrading organisms Ideonella sakaiensis from plastic-contaminated environments (soil, water) to isolate genes involved in plastic degradation.
- DNA Extraction: Extract genomic DNA from these samples using chemical methods or extraction kits, focusing on genes responsible for PET degradation (such as PETase and MHETase).
Primer Design:
- Target Identification: Using known sequences of PETase and MHETase genes, design specific primers that bind to the target regions.
PCR Amplification:
- Gene Amplification: Mix extracted DNA with primers, nucleotides, buffer, and DNA polymerase to perform PCR. PCR cycles will amplify the PETase and MHETase genes, creating multiple copies for cloning.
Detection and Purification:
- Confirmation and Purification: Check PCR products via gel electrophoresis. A distinct band at the expected position confirms successful amplification. Purify these gene fragments to prepare for insertion into yeast cells.
2Gene Optimization for Enhanced Expression
Codon Optimization
- To ensure efficient expression of PETase and MHETase in Saccharomyces cerevisiae, codon optimization is essential, as yeast may not naturally favor all codons present in the original genes. Using bioinformatics software (Such as GeneOptimizer), the gene sequences are modified to replace low-frequency codons with those preferred by yeast. This process enhances compatibility with yeast’s translation system, leading to more efficient protein synthesis and higher yields of PETase and MHETase, which improves the plastic-degrading efficiency of engineered yeast.
Regulatory Elements
- A strong promoter - GAL1 promoter is used to drive high levels of gene expression. The promoter is a crucial regulatory element in the DNA sequence that attracts RNA polymerase, controlling the rate of gene transcription. In this project, a strong promoter ensures that PETase and MHETase genes are transcribed at high levels, producing sufficient enzymes for effective PET plastic degradation.
- Additionally, enhancer sequences can be added near the promoter to further increase its activity, enhancing the transcription rate and maximizing enzyme yield. A terminator sequence helps stabilize mRNA after transcription is completed, preventing resource wastage from extended transcription.
3Select a host for production
Why Choose Saccharomyces cerevisiae
- Saccharomyces cerevisiae (yeast) is an ideal production host due to its ease of cultivation, genetic manipulability, and well-established use in industrial fermentation. Unlike bacteria, yeast can be cultured on a large scale with minimal cost, and its genetic structure allows for efficient gene insertion and expression. By genetically enhancing yeast to produce PETase and MHETase, we create a highly adaptable and scalable solution for plastic degradation, capable of handling industrial applications.
Other Host Characteristics
- Yeast can thrive in a variety of environmental conditions and has high environmental adaptability, making it suitable for diverse plastic-polluted settings, such as soil, freshwater, and marine environments. Using yeast as the production host ensures it can be applied to real-world environmental applications, providing a sustainable solution for large-scale plastic degradation.
4Genetic Engineering of the Host
Plasmid Construction
In genetic engineering, a plasmid is a small, circular DNA molecule independent of the host cell’s chromosome and is commonly used as a vector to carry foreign genes. For this project, the constructed plasmid contains optimized PETase and MHETase genes, which are essential for breaking down PET plastic into environmentally safe byproducts. These genes are codon-optimized to ensure high expression levels in Saccharomyces cerevisiae, aligning with the yeast’s translational machinery for efficient protein production.
Gene Structure and Selective Marker
- PETase and MHETase Genes: The core components of the plasmid are the PETase and MHETase genes. PETase breaks down PET plastic into an intermediate compound, MHET, which is further degraded by MHETase into simpler molecules. Codon optimization has been applied to these genes to match S. cerevisiae’s codon preferences, ensuring that PETase and MHETase are expressed at maximum efficiency within the yeast cells.
- Antibiotic Resistance Gene: The plasmid also includes an antibiotic resistance gene, such as Ampicillin (Amp) or Kanamycin (Kan), which acts as a selectable marker. This gene enables yeast cells that successfully acquire the plasmid to survive in environments containing the corresponding antibiotic, while cells without the plasmid cannot grow. This selection process allows researchers to effectively identify and isolate transformed yeast cells, simplifying the screening of successful transformations.
Strong Promoter and Expression Control
- To ensure robust expression of PETase and MHETase in yeast, a strong promoter, such as the GAL1 promoter, is used in the plasmid design. The promoter is a regulatory element positioned upstream of the target genes, attracting RNA polymerase and initiating transcription. The GAL1 promoter, a well-established strong promoter in yeast, drives high transcription levels, ensuring that sufficient quantities of PETase and MHETase are produced to meet the demands of plastic degradation.
- Enhancer sequences are added near the promoter to further boost transcription, while a terminator sequence downstream of the genes helps stabilize mRNA and prevents unnecessary transcription. This well-coordinated design optimizes gene expression, maximizing enzyme production and enhancing the plastic degradation potential of the engineered yeast.
Preparing the Plasmid for Yeast Transformation
- Once the plasmid’s gene structure and regulatory elements are optimized, it is ready for insertion into Saccharomyces cerevisiae. The plasmid can integrate into the yeast genome or exist as an episome (an independently replicating DNA molecule), allowing the modified yeast cells to maintain continuous expression of PETase and MHETase. This plasmid construct enables S. cerevisiae to efficiently produce enzymes for plastic degradation, providing a scalable solution for environmental applications.
Transformation (Gene Insertion)
- Transformation Technique: In this project, we use lithium acetate transformation, a widely used method for introducing foreign DNA into yeast cells. This technique alters the yeast cell membrane's permeability, enabling the plasmid DNA to enter the cell. Yeast cells are treated with lithium acetate and polyethylene glycol (PEG) to increase membrane permeability, followed by a heat shock to facilitate plasmid entry.
- Gene Integration: Once inside the yeast cell, the plasmid either integrates into the yeast’s genomic DNA or remains as an independent episome. Regardless of its form, the strong promoter ensures that PETase and MHETase are continuously expressed at high levels, enabling the yeast to degrade plastic effectively.
Screening and Verification
- Selection: After transformation, the yeast cells are plated on a medium containing the antibiotic corresponding to the resistance gene on the plasmid. Only cells carrying the plasmid survive and grow on this medium, allowing easy identification of successfully transformed yeast strains.
- Verification: Once selected, these yeast strains are further verified through PCR and enzyme activity assays. PCR confirms the presence of PETase and MHETase genes within the yeast cells, while enzyme assays assess the activity of these proteins, confirming that the engineered yeast strains possess the desired plastic-degrading capabilities. This verification ensures that the genetically modified yeast will function as intended in the degradation process.
5Large-Scale Cultivation and Production
Bioreactor Cultivation
- A bioreactor provides a controlled environment for yeast growth, allowing precise adjustment of temperature, pH, and oxygen levels. This setup ensures optimal enzyme secretion for PET degradation. Real-time monitoring and control enable consistent and efficient large-scale production, making it viable for industrial applications.
- The advantage of a bioreactor is that it allows real-time monitoring and adjustment of culture conditions, ensuring the bacteria remain in ideal conditions throughout the entire cultivation process, thereby achieving consistent and stable degradation. This control capability is crucial for scaling up to industrial production.
PET Induction
- PET plastic fragments are added to the culture medium within the bioreactor, activating enzyme expression in the bacteria. PET serves as the substrate, guiding the engineered strains to produce large amounts of PETase and MHETase enzymes, which then begin breaking down the plastic.
- Over time, PET plastic is gradually converted into terephthalic acid (TPA) and ethylene glycol (EG), achieving complete plastic degradation. Through this induction process, the degradation efficiency can be optimized, and plastic pollution in the environment can be significantly reduced.
6Recovery and Purification of Byproducts
Byproduct Monitoring
- Liquid chromatography or gas chromatography techniques are used to analyze byproducts in the culture medium, monitoring the concentrations of TPA and ethylene glycol (EG) to confirm complete degradation. These analytical techniques are highly sensitive, enabling precise detection and quantification of degradation products to ensure no residual plastic remains.
Separation and Collection
- After the degradation process is complete, bacterial cells and degradation products are separated using filtration or centrifugation techniques. Filtration effectively removes bacterial cells, leaving only TPA and EG as degradation products.
- The collected TPA and EG can be further purified and used as raw materials in other industrial processes, achieving byproduct recycling and resource reuse. This approach makes the degradation process both environmentally friendly and economically efficient.!
7Testing and Safety Assessment
Efficacy Testing
- Environmental Conditions Testing: The degradation efficiency of engineered yeast is tested under various temperatures, pH levels, and pollutant concentrations, simulating different environmental conditions.
- Performance Validation: These tests confirm the strain’s stability and effectiveness in real-world applications, ensuring consistent degradation of PET plastic.
Environmental Safety Assessment
- The behavior of the engineered strain is observed in simulated natural environments to ensure it does not negatively impact ecosystems or human health. These assessments include evaluating the strain’s potential effects on other microorganisms, soil, water bodies, and human health.
- Through a series of ecological evaluations, researchers confirm that the engineered strain’s degradation activity remains within safe limits, avoiding any ecological imbalance. Completion of safety assessments demonstrates that this strain complies with environmental and human safety standards, supporting its potential for wider application in real-world scenarios.
MCB340 Assignment 3 - Xiuchen Lu